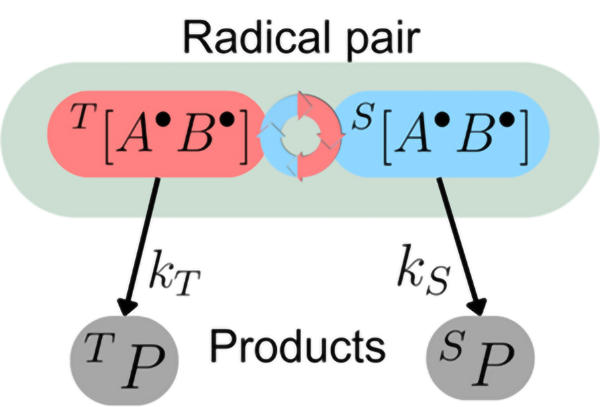
In the enchanting world of nature's mysteries, many migratory animals possess a remarkable compass-like sense known as "magnetoreception." This extraordinary ability enables them to embark on epic journeys guided by the Earth's magnetic field. Imagine songbirds taking to the skies, their internal compasses attuned to the subtle dance of our planet's geomagnetic forces. Recent behavioural experiments have unveiled a fascinating facet of this avian talent: some songbirds possess a light-activated magnetoreception. What makes this discovery even more captivating is that these birds rely on something other than traditional magnetic minerals for their celestial navigation. Instead, they seem to wield a chemical compass, a marvel rooted in quantum physics. At its core lies the enchanting concept of the "unstable radical-pair reaction," where two molecules come together to share a pair of electrons with correlated spin states. This reaction, like a finely tuned instrument, is exquisitely sensitive to the Earth's gentle magnetic whispers and can function as a celestial compass, allowing the birds to gauge their orientation with respect to the geomagnetic field.
With advanced computational techniques, we embark on a thrilling journey to explore the intricate dynamics of these chemical reactions. We study how they unfurl their secrets under ever-changing environmental conditions. At the heart of this research lies the promise of unlocking the hidden intricacies of our feathered friends' celestial navigation, bringing us one step closer to comprehending the mesmerizing mysteries of the animal kingdom.