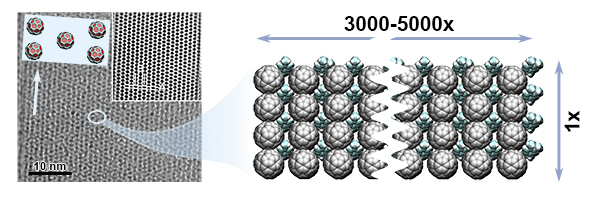
The growth of one-dimensional (1D) nanocrystals represents an important research topic in crystal engineering for nanotechnology. The growth of 1D fullerene (C60) nanocrystals (or nanowires) has proven to be of considerable scientific and technological interest because of the properties associated with the low-dimensionality, quantum confinement effect, and potential electronic, magnetic and photonic applications. In this research project, we investigate the growth process of these unique one-dimensional nanowires made of a C60. A fascinating aspect here is that these nanowires can be grown without any metal catalysts, which is typically required in other methods. By studying the arrangement of molecules in these nanowires and how they bond, we aim to understand how to control their growth and shape. Our results have indicated that these nanowires can become insoluble in common solvents over time, leading to enhanced thermal stability. The project delves into the chemistry and physics of these nanowires to unlock their potential for various applications in nanotechnology, offering valuable insights into their unique properties and growth mechanisms.