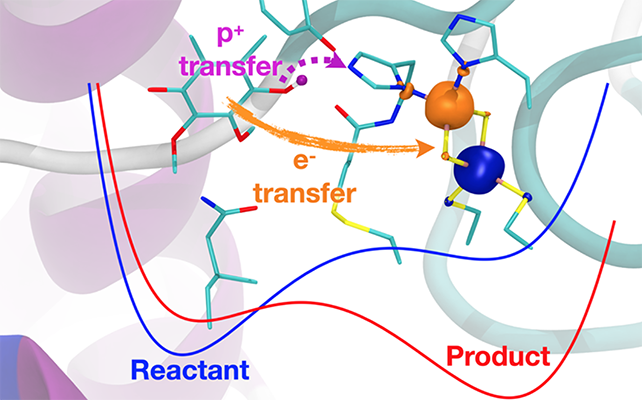
The cytochrome bc1 complex is a transmembrane enzymatic protein complex that plays a central role in cellular energy production and is present in the photosynthetic and respiratory chain organelles. Its reaction mechanism is initiated by the binding of a quinol molecule to an active site, followed by a series of charge transfer reactions between the quinol and protein subunits. Previous work hypothesized that the primary reaction was a concerted proton-coupled electron transfer (PCET) reaction because of the apparent absence of intermediate states associated with single proton or electron transfer reactions. In this project, we investigate the kinetics of the primary bc1 complex PCET reaction with a vibronically nonadiabatic PCET theory in conjunction with all-atom molecular dynamics simulations and electronic structure calculations. Specifically, within this multiscale theory, we treat the electrons and transferring proton quantum mechanically and derive the underlying parameters from large-scale, all-atom MD simulations, which provide the reorganization energy and reaction free energy. Cytochrome bc1 complex serves as one characteristic example of a PCET-driven system. At the same time, our studies aim toward the development and application of a general strategy for describing PCET reactions in bioenergetic systems.